3 4 Racing Cam For A 454 Big Block Youtube
- 3 4 Racing Cam For A 454 Big Block Youtube
- 3 4 Racing Cam For A 454 Big Block Youtube Chrome
- 3 4 Racing Cam For A 454 Big Block Youtube Download
- 3 4 Racing Cam For A 454 Big Block Youtube Channel
The following specs apply to:
- Gen IV, Big Block Chevy
- Mark V, Big Block Chevy
Richard Holdener swaps a cam in a 454. This video shows you the step by step instructions so you can too.Subscribe to our YouTube channels:COMP Cams http://w. Almost none of the parts carry over from previous engines. The camshaft is a steel roller cam, approximately 4” shorter and.300” larger in diameter, so there should be no problem with misapplications. Big Block -uses “11” Prefix, Gen VI Big Block -uses “01” Prefix, 8.1L Big Block -uses “46” Prefix.
Fastener Type | Torque Specs |
Main Cap Bolt (396-427, 2-Bolt) | 95 ft.-lbs. |
Main Cap Bolt (396-454, 4-Bolt, Inner and Outer) | 110 ft.-lbs. |
3/8 in. Connecting Rod Bolt | 50 ft.-lbs. |
7/16 in. Connecting Rod Bolt | 67-73 ft.-lbs. |
Cylinder Head Bolts, Long | 75 ft.-lbs. |
Cylinder Head Bolts, Short | 65-68 ft.-lbs. |
Screw-In Rocker Arm Studs | 50 ft.-lbs. |
Intake Manifold Bolts (Cast Iron Head) | 25 ft.-lbs. |
Oil Pump Bolt | 65 ft.-lbs. |
Cam Sprocket Bolts | 20 ft.-lbs. |
Harmonic Damper Bolt | 85 ft.-lbs. |
Flywheel/Flexplate Bolts | 60 ft.-lbs. |
Pressure Plate Bolts | 35 ft.-lbs. |
Bellhousing Bolts | 25 ft.-lbs. |
Exhaust Manifold Bolts | 20 ft.-lbs. |
Notes
- These specs are for stock-type bolts with light engine oil applied to the threads and the underside of the bolt head. Moly and other lubes offer reduced friction and increased bolt tension, which will affect the torque figure. If you use aftermarket performance bolts like ARP's, you should follow the recommended torque specifications.
3 4 Racing Cam For A 454 Big Block Youtube
Although I have written about the determination of precise valve-event timing on numerous occasions, it’s still a subject shrouded in mystery for even the majority of pro engine builders. In an effort to save all big-block owners/builders from installing the wrong cam, I am going into the science behind valve events in greater depth. After you have absorbed this information, you will know more on the subject than the typical cam tech guy whom you might otherwise have called. I am doing this for two reasons: First, it will be a really great help to your engine spec’ing and building skills. Second—and be sure to really take note here—you would have otherwise bought the wrong cam for the application.
This Tech Tip is From the Full Book, CHEVY BIG-BLOCKS: HOW TO BUILD MAX PERFORMANCE ON A BUDGET. For a comprehensive guide on this entire subject you can visit this link:
LEARN MORE ABOUT THIS BOOK HERE
SHARE THIS ARTICLE: Please feel free to share this article on Facebook, in Forums, or with any Clubs you participate in. You can copy and paste this link to share: https://www.chevydiy.com/chevy-big-block-performance-camshaft-and-valvetrain-events/
Fig. 9.1. The traditional way to optimize a cam for a given engine spec is typically to spend considerable time and test a number of cams to find the best one. With experience, a good engine builder can get very close after, on average, about six cams. All this costs a lot of time and money. You want to get the cam spec really close to optimal the first time around and without the normally mandated use of a dyno.
For some reason, cam-spec’ing skills for a big-block Chevy are in short supply. The result is that about 95 percent of cams are off the mark. And they are off to the tune of 25 to as much as 75 hp. I need to make it clear here that a cam with the wrong opening and closing events costs exactly the same as a cam with the right events. What you learn in this chapter and Chapter 10, Valvetrain Optimization, is worth many, many times what you paid for this book.
A Few Basics
Before proceeding, I need to make sure you are aware of some very important induction facts that are not common knowledge. First, air is heavier than you might think. A 100-foot cube weighs 38 tons. This fact should lead to placing more importance on port velocity. Air is a gas, and once moving, it takes quite a bit to stop it. Port velocity for the purpose of ramming the cylinders at the end of the induction stroke can be way more effective than many cylinder head pros recognize.
Second, you need to drop the notion that the piston is the primary source of air demand during the induction cycle. (I can take port and in-cylinder pressure measurements on my own Chevy big-blocks.) Let’s forget the all-out race units here, and look at what you are probably building: a good, stout street/strip engine.
On such an engine, the piston suction from the downward movement in the bore generates, at peak demand, about 1 psi at about 500 rpm prior to peak power. At that same RPM, a well-designed header system that was scavenging produces suction of 11 psi at the exhaust valve while the piston is at TDC. About 8 psi of it traveled past the exhaust valve to the cylinder. In simple terms, the exhaust drew on the intake valve and port eight times harder than the piston going down the bore. With the piston parked at TDC the port velocity into the cylinder was almost 100 mph!
Does any of this fit your conception of the intake stroke? Most likely not. With that in mind, clear your thoughts of preconceived ideas concerning what it takes to open and close the valves at the right time for your specific engine. Also, the closing of the intake is not the most important part of valve events so throw that popularly held notion out as well.
As a matter of reference, when I talk about duration with solid lifters, I am typically dealing with the duration at lash referred to as “off-the-seat” duration. Depending on the tappet ramp, this is typically 10 to 14 degrees longer than the 0.020-inch tappet lift duration most often used by cam companies.
The Importance of Overlap
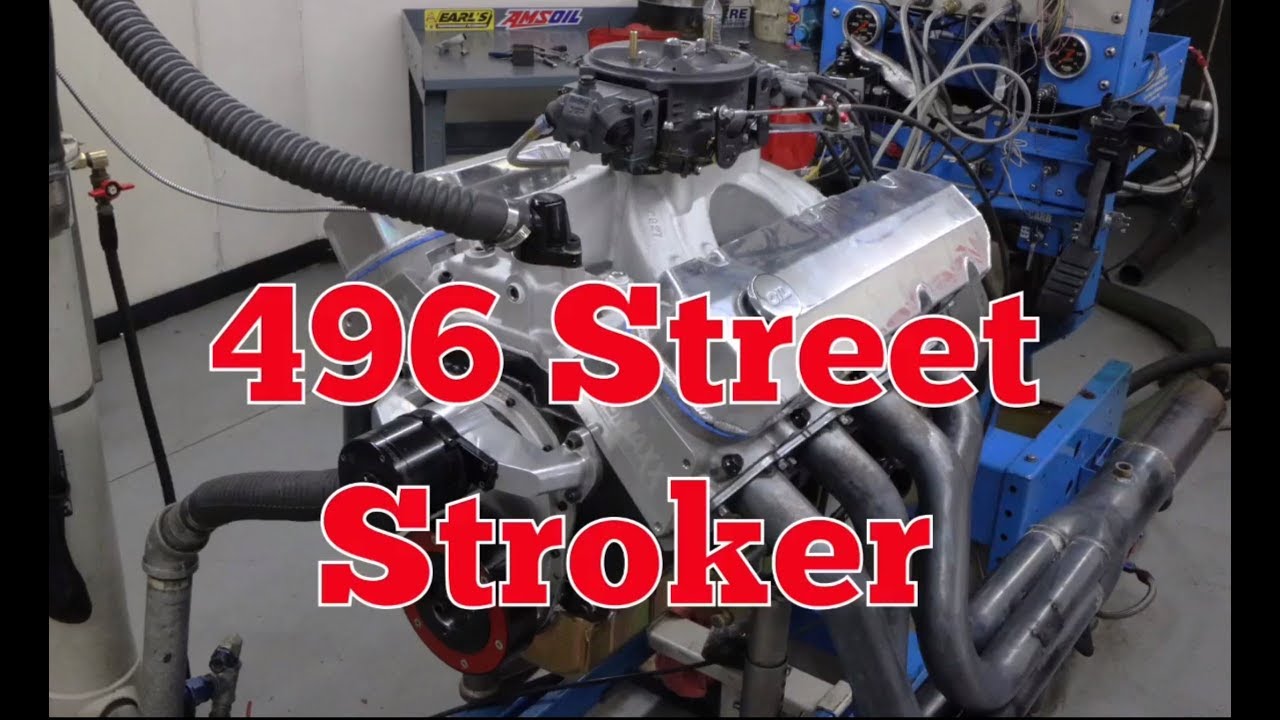
The events that occur in the exhaust/intake overlap period are of utmost importance. They take place before the piston really gets underway down the bore on the intake stroke. From many hours studying pressure traces of a variety of engines, one thing becomes clear, and it is the very point that makes the overlap the most important valve event, rather than the often-supposed intake closing point. This is an important point you should never let slip from your mind when it comes to valve events for maximum output.
Fig. 9.2. Getting the overlap triangle right is the key to producing power and torque from a big-block Chevy. Doing so involves, among other things, selecting the right duration and the right cam lobe lift and valve lift via the subsequent rocker ratio selection.
Pressure studies have revealed that if the cylinder-filling process is not optimized in the first half of the induction cycle, nothing can be done to redeem it in the second half. A 5-degree error in the amount and positioning of the overlap triangle around TDC has at least double and maybe triple the impact on output that a similar error has on the closing of the intake. In simple terms, if the first half of the induction cycle is below par, the entire induction cycle is even further away from optimal.
Overlap Functions
It should now be clear that the most important factor toward both big torque numbers and top-end power is the way you deal with over-lap. The “overlap triangle” (Figure 9.2) must be positioned at about TDC so it experiences the optimal benefits of the very strong exhaust extraction pulse that a performance exhaust system can deliver. Anything short of optimal is, in effect, like compromising an otherwise free induction event. In addition, this is critical in the case of an under-valved big-block; you need to get the intake valve underway well in advance of the piston demand. This produces a higher lift earlier on in the piston phase of the induction cycle and thus partially compensates for an intake valve that is too small for the job.
When spec’ing a cam, you are seeking to apportion various amounts of the opening period of both intake and exhaust to specific areas within the entire induction, exhaust, and power events that the piston goes through. In other words, for a given intake and exhaust opening event you have to decide how much should occur during the over-lap, how much should occur on the intake up to the closing point after BDC, how many degrees before BDC you should open the exhaust, and so on. Understandably, this may seem like a lot to grasp; let me simplify.
Fig. 9.3. The most important cam parameters are the intake (1) and exhaust (2) centerlines, the LCA (6) and its relation to cam advance (A) and retard (R). Remember, cam advance/retard (7) assumes the crank is held still during cam adjustment and that is why the advance and retard appears the wrong way around. The intake duration (3) and the exhaust duration (4) positions on the cam are controlled by the LCA (6), and this positioning, along with the duration, dictates what the overlap (5) is. For a Chevy big-block, the lobe lift of the intake is a very important factor. Because the cam is physically bigger (1.950-inch journal diameter) than a small-block (1.872-inch journal diameter), the lobe can, for a given duration, have a higher lift. However, be aware that many cam companies use the same profiles for a small-block. If the profile is designed especially for a big-block and fully utilizes the extra diameter, it can result in a profile with as much as 0.012-inch greater lift.
If you can come to a viable decision on amount of overlap and cam-shaft lobe centerline angle (LCA; also known as lobe separation angle, or LSA), the cam spec will be pretty much locked in (Figure 9.3). To see how this works, just check out the example in Figure 9.5. Here you see that if you are using a single-pattern cam, and the overlap and LCA required is 80 and 106 degrees respectively, then the duration cannot be anything other than 292 degrees.
Overlap Considerations
Here’s an important question: How much overlap does the engine need for a given application? The application dictates the answer, and the fact is that the more racy the application, the more overlap is needed. But there is an important factor in addition to this basic assumption. If you use the existing head specs, larger displacement engines using a given set of heads can tolerate more overlap for a given idle quality and low-speed output.
For example, let’s consider the smallest big-block that I have in my arsenal of test engines: 434 inches. This engine has a cam with 83 degrees of overlap, and as such, it does not idle very well. However, 83 degrees of over-lap for a 632 is not excessive overlap and idle quality is more than acceptable for a street/strip engine.
At the end of the day, performance at low speed is very much a question of how quickly the intake charge travels in only one direction as RPM increases. Any rough-running and/or reduced low-speed torque attributes are typically the result of exhaust pollution of the intake charge during overlap (rough idle) and reversion due to adverse pressures causing the gases to travel in the opposite direction they are supposed to; this reduces low- speed torque.
Here is a classic example: At low RPM the piston pushes some of the just-inducted intake charge back out of the cylinder through the still-open intake valve as the piston starts up the bore on the compression stroke. This continues until the intake charge velocity gains sufficient kinetic energy to pile back into the cylinder.
Fig. 9.4. This chart does not provide the complete answer to the selection of a viable overlap requirement because it is influenced by the amount of lobe lift the cam has and how aggressive it is. With typical profiles, it is in the ballpark of overlap required for a Chevy big-block. To determine what you need, first follow the top line across the chart until your engine’s cubic-inch spec is reached. Next, go down the left-hand RPM scale until you are at the RPM you want the cam to reach. Finally, go across to the right until the RPM line intersects with the cubic-inch column. That intersection point is the overlap required. Be sure to use at least the minimum compression ratio recommended (far right-hand column). Note: These overlap figures are for solid cams as determined by the 0.020-inch tappet-lift figures from the cam manufacturers’ catalogs. Hydraulic cams are typically measured at 0.006-inch tappet lift for the advertised duration, so add 10 degrees to the overlap calculated by this chart.
Fig. 9.5. Shown here is a cam of some 270 degrees duration. The key to getting the most from any given duration is to apportion the amount of intake valve opening before TDC (magenta in top illustration) with that occurring after BDC (green). It is very important that the intake valve starts far ahead of the piston-driven part of the induction stroke because the intake valve is too small to be able to allow good filling unless it is opened faster and farther to compensate. Closing the intake too far after BDC inevitably leads to excessive reversion at low RPM and this can cut low-speed torque considerably. If the intake valve is opened too late, a delayed closure will not compensate. Because of its short rod/stroke ratio, the piston travel (PT) up from BDC is measurably less than for a long-rod engine. This means you can hold the intake valve open slightly longer before the effects of reversion become an issue.
Fig. 9.6. The overlap triangle area is a combination of valve lift and degrees of duration by each valve during the overlap period. It is better to develop the overlap area by means of valve lift rather than degrees of overlap duration. High-lift rockers, such as these from PRW, are almost 1.9:1 and allow a shorter cam to produce more top end without as much loss in idle quality and low-speed output.
Delayed intake closure means that the cylinder traps less charge than it could have otherwise. As a consequence, low-speed torque is greatly reduced. In the final analysis, it’s all about gas speeds. What you see in practice is that, for a given over-lap or delayed valve closure, a barely acceptable idle at 1,050 rpm with a 400-ci engine results in a 680-rpm idle for a 600-ci engine.
You must determine how much overlap you need to build into the cam spec. Figure 9.4 is your starting point. This assumes a typical 2.3-inch intake valve. If the valve is bigger, the amount of overlap needs to be reduced for any given low-speed requirement. If a smaller valve, such as the 2.190-diameter is used (many entry-level heads have them), a little more overlap is required. For every 0.050-inch difference in valve size from 2.3, you need about 3 degrees up or down to get the job done.
So far, I have discussed overlap only in terms of degrees of crank rotation, but that’s just half the story. The other half is the amount of lift the valves experience during that overlap period.
The best way to think about over-lap is to visualize the triangle shape, which is produced at about TDC on the cam event diagram. That, in degrees and lift, is what finally counts. If idle quality is the issue, then, for a given overlap triangle area, the negative effect of overlap is greater than opening lift at TDC. So, if you want the most effective over-lap (one for which you take the least idle-quality and low-speed impact), you are better off reducing the number of degrees in favor of faster valve action. For a given overlap area, developing it by means of more lift and fewer degrees is preferable.
Another factor is the intrusion of the exhaust into the intake part of the overlap. This has a greater negative impact on idle than does the intake. You can do a simple test by lashing the exhaust very loosely and then comparing idle quality with an equally loose intake. Lashing the exhaust approximately 0.015-inch looser than necessary while holding the intake lash to spec has a greater effect on smoothing the idle than when the same test is done with the intake while holding the exhaust to spec.
3 4 Racing Cam For A 454 Big Block Youtube Chrome
LCA Selection
As I am a one-man engine consulting business, it is important for me to achieve optimal cam specs very quickly. Many of my clients would certainly balk if asked to pay for buying and testing a dozen or more cams through an engine just to find out what worked best. As it happens, many clients are cam companies, and the opportunity to test hundreds of cams in multiple engine types allows me to unravel the seemingly mysterious life of a camshaft.
In 1997, I put thousands of hours of cam test data accumulated over 35 years to good use. After working on it for a year, I had a functional computer program that formed the basis of my current Cam Master program, which is not available to the general public. This program proved to be extremely accurate at predicting valve events, and consequently, LCA dyno testing multiple cams is virtually a thing of the past.
The following discussion gives you concise information on which to base the selection of the LCA for your engine build as opposed to choosing something roughly similar built by Joe Blow down the road. Sure, he may have had “good luck” with the cam he used, but if you are going to rely on luck rather than technology, there was no point in buying this book in the first place. Let’s explore why a certain spec of engine prefers one LCA to another. I have stated that you need to divide up the valve opening events so that they are optimally assigned. That is, you want just the right amount of the available intake duration to happen before TDC (in the overlap period) and after TDC (during the delayed closer part of the complete valve opening and closing event). The same goes for the exhaust. Getting this balance to an optimum entails having the correct LCA. Also remember that pressure tests of the intake, combustion chamber, and exhaust revealed that it was paramount that the intake event be optimally initiated.
Fig. 9.7. To calculate the required LCA specifically for a Chevy big-block 24-degree head, divide the cubic inches of one cylinder by the valve diameter. Look that up on the scale to the left and then go across to the right until the red line is intersected. Now drop down to the bottom scale. This is the LCA required. This chart is based on 1.8 and 1.7 rocker ratios for the intake and exhaust, respectively, and a compression ratio between 10:1 and 11:1. If the compression ratio is above 11.5:1, the LCA needs to be corrected.
Fig. 9.8. Illustrated here is why vacuum drops as cam overlap increases. A V-8’s induction cycles are 90 degrees apart. This means that when one piston is at TDC in the overlap period (left-hand cylinder), another is halfway down the bore on its induction stroke. The small blue circle represents the area available at the nearly closed carb butterflies. The larger red dot represents the flow area from the exhaust system through the exhaust valve into the cylinder to the intake valve and into the intake manifold. The smaller red dot is for a moderate- performance street cam while the larger red dot is for a big-performance street cam. You can see how much easier it is for the cylinder, on its induction stroke, to draw on exhaust rather than draw through the carb restriction.
After crunching the data from the very substantial number of cam tests available, it became apparent that the LCA was directly linked to the ratio of the intake/exhaust flow of the valves at low lift to the engine’s displacement. The greater the initial flow rates per cube, the wider the optimal LCA became and visa versa. Testing further demonstrated the intake flow was by far the most influential.
An example here serves to illustrate the trend. Let us assume, by virtue of numerous tests and a substantial degree of luck, you have the LCA correct for a given combination of heads and cylinder displacement. The low-lift flow is increased. When this is the case, the optimal LCA gets wider because of the flow capability of the intake during the lift experienced during overlap. When the number of cubes that the heads have to service is increased, the flow becomes worse for each cubic inch. The remedy is to tighten the LCA.
In practice, this plays out as follows. You buy a set of heads to go on your 468 and by sheer chance the cam you have is right on the money for the head flow/displacement combination. Down the road, you decide to rebuild your engine with a 1/2-stroker crank to make it a 525. Without the aid of this book, many engine builders would retain the cam used for the original 468, especially if the combination worked well.
However, if the cam’s LCA was correct for a 468, it typically needs to be tightened by at least 2 to 3 degrees to be optimal for a 525, assuming the same heads are used. Also assuming a 10.5:1 CR for the 468, the optimal LCA would typically be 107 to 108 degrees. But the heads now have to feed extra displacement and this requires the intake valves to open sooner, so that they have more of a head start on the piston-driven phase of the induction event.
Also, if the compression ratio remained unchanged, the combustion chamber volume is bigger. This means the amount of spent gas the exhaust has to extract at and around TDC is greater so the overlap triangle needs to be proportionally bigger. The result is that the 525 build typically requires an LCA of about 105 degrees.
Flow Figures
To establish the optimal LCA, the cylinder heads’ flow figures, starting at 0.050 lift, are needed. For the record, using 0.200 as a starting point for the measurement of flow curves is based on the erroneous assumption that the flow under that level of lift is irrelevant. Nothing could be further from the truth! Low lift flow is a prime factor affecting the selection of an optimal LCA. This means that if you are dealing with flow figures to spec out the cam, you need to know what the low lift flow is.
At this point you may be thinking that all of this is well and fine, but you do not have any flow figures for your heads or access to a bench to get them. There is another solution: Some very impressive results using intake valve dimensions instead of flow-through to determine the final answer are possible. Although this method is less accurate than using a flow bench, it is far better than wading through a cam catalog for help to make the choice. When using this valve-sizing method, output can typically improve by 30 ft-lbs and 30 hp or more.
The cylinder you are trying to fill only recognizes flow. It does not recognize valve diameter or lift at any time during the lift event. It is important to keep that in mind when considering the events taking place within the engine’s induction system. But to make a correct choice, another factor comes into play here: The low-lift flow is influenced only by the valveseat design. There are usually only minimal differences from one high-quality valve job to another. That holds true even if there is a big difference in high-lift flow, due to port design, later in the opening event. Therefore, using the intake valve size as the determinant variable still yields results far better than even the most well-educated guess from an accredited expert engine builder.
At the end of the day, the optimum LCA, when graphed out as the engine’s displacement versus peripheral length of the intake valve and consequently its diameter, produces a nearly straight line. Exactly where on the graph that line falls also depends on the valves’ inclination because this has a measurable effect on cross flow during overlap. The more angled the valves, the more you have to compensate by widening the LCA, and therefore reducing the amount of overlap for a given duration.
The graph in Figure 9.7 already compensates for the big-block’s compound valve angles. This graph also assumes that the engine is equipped with a decent exhaust and intake system. If the intake and exhaust are good, for a 24-degree Chevy big-block with a compression ratio approximately between 9.5 and 11:1, Figure 9.7 computes the LCA required very precisely.
LCA Compression Correction
In Figure 9.7, the LCA can be determined for engines with a compression ratio between 9.5:1 and 11:1. Although the low-lift flow in relation to displacement is the primary influence, the compression ratio’s influence comes in at a close second place. The compression ratio can have a significant effect on the optimal LCA and you need to recognize this important factor. (The strongest criticism of the accuracy of Cam Master’s output comes from this.) For a typical street engine, most of the cam LCAs calculated by Cam Master usually produce an LCA of 106 to 108. These LCAs are tight compared to those used for a Pro Stock engine, which are typically 114 to 116 (as predicted by Cam Master). This is often cited as proof that Cam Master calculations must be incorrect.
However, you must not overlook the fact that a hopped-up street 10:1 big-block bears little resemblance to a Pro Stock engine regardless of the fact that they may share the same Chevy logo. You must recognize that more valve area per cube and a compression ratio well in excess of 16:1 make a huge difference in what is required for an optimum LCA.
In an analysis of compression ratio versus LCA remember that the exhaust needs to scavenge just a little more than the volume contained in the combustion chamber. Over-scavenging impacts fuel efficiency, and this is a concern for a street engine. On the other hand, a certain amount of over-scavenging is good for an all-out drag race engine in which fuel economy is not a concern. For a 17:1 CR 500-ci Pro Stock engine the total combustion chamber volume is 63 cc. For a 10:1 556-ci engine the chamber volume is 128 cc. That is a big difference. As a result, to scavenge a chamber of 128 cc the overlap area needs to be substantially greater in proportion to the overall duration. This means that a lower compression engine must have a tighter LCA.
There is a lesson to be learned from this discussion in addition to how the compression ratio may effect what your engine needs. That lesson is: Never take a single feature of an extremely well developed engine and apply it to your engine because it almost certainly won’t work in the way you expect.
In addition, the proportion of the valve opening duration that occurs during the overlap needs to be less for reasons other than the chamber volume compared to the overall cylinder volume (i.e., the compression ratio). This is because, as the compression ratio increases, the exhaust velocity at the end of the exhaust stroke event increases as the piston approaches TDC. This occurs because even though the piston is slowing toward the top of the stroke, the rate of volume change for the remaining volume increases as the compression ratio increases.
Although one factors tends to cancel the other, a net increase in port velocity occurs over the last few degrees before TDC. This increase in velocity improves the scavenging effect so it takes slightly less overlap to get the job done than would have otherwise been the case.
Figure 9.7 provides the LCA required for a typical street compression ratio, which is between 9.5 and 10.5:1. In practice, the engine delivers good results to about 11 to 11.5:1 CR, but above that a compression ratio compensation of the LCA is definitely needed for best results. If you are targeting more compression, the LCA needs to be spread by 2 degrees for every ratio higher than 11.5:1. Now, consider this example: an all-out race engine with 582 ci and a 15.5:1 CR. The compression ratio is four ratios higher than the 11.5:1 base number on Figure 9.7, so you need to spread the LCA by 8 degrees. The chart shows a 104-degree LCA for a street-engine compression ratio. Factoring in the additional 8 for the high compression yields a required LCA of 112 degrees. This, in practice, is just where an engine with a 2.3-inch intake valve and 24-degree heads ends up.
To confidently determine the LCA of race engines, you need to know that at lower compression ratios 1 degree off the optimal LCA can be quite substantial. If the LCA is too wide by just 1 degree it can, for a typical 496, result in the output falling short to the tune of some 10 to 15 ft-lbs and a similar amount in horse-power. If the cam selection turns out to be 1 degree too tight, the performance down the drag strip is virtually unchanged, but the idle quality suffers, as does the torque right off idle, to the point that the engine really comes “on-the-cam.” That point is usually about 700 to 1,000 rpm before peak torque RPM.
If track performance is your goal, it is better to be 1 degree too tight than 1 degree too wide. If street manners and idle are important, the reverse applies. If you are dealing with roller cams that have lower initial acceleration, the effect of an LCA that is 1 degree too tight is almost lost and its effect is negligible. If you are using a short-duration cam, which is less than 270 degrees of off-the-seat duration, for strictly the best all-around street performance, erring on the tight side for a LCA selection has only a minor effect on low-speed operation. Flat-tappet cams have higher initial acceleration, and thus are more sensitive to being 1 degree too tight as opposed to 1 degree too loose.
When the compression ratio nears 13.5:1 and higher, the LCA for optimum output becomes less critical than for a street engine in the 10:1 range. I saw the reduced sensitivity to LCA changes when testing cams in a 16.5:1 500-ci Pro Stock engine. LCAs from 112 to 116 were tested in 1-degree increments. Over the RPM range used on the track the output only changes about 15 hp in 1,400 overall hp.
Optimal LCA
Other than the effects of valve size versus displacement and the compression ratio, there are other influences that need attention. One is the effect that the intake rocker ratio has on the selection of a nearly optimal LCA. Remember, the intake valve does not know how much lift has been imparted to it.
In practice, the cylinder only recognizes the flow available at any moment in time. A valve that is opened faster looks the same as a bigger valve or a valve having greater flow efficiency while being opened at a slower rate. If the cam LCA is optimal, using a higher ratio rocker means you need to spread the LCA.
Remember that it is not the stated overall rocker ratio that affects the LCA. It is the ratio that exists as the valve comes off the seat to the point that the overlap period ends. (See Chapter 10, Valvetrain Optimization.)
Just because a rocker has 1.8 written on it does not mean it is actually 1.8. If you use the valvetrain advice given within these pages, the chances are you are using as high a ratio rocker as possible on the intake. For that reason, the curve on the LCA chart in Figure 9.7 is specifically for a 1.8/1.7 intake/exhaust rocker ratio combination.
The rod/stroke ratio is another factor that has an effect on camshaft specs. If you consider all engines, the geometry needed by long-rod engines is different from that needed by short-rod engines. However, in dyno tests when there’s been a 1-inch change in rod length, the optimal LCA changes only marginally. With a big-block Chevy, it’s all about extracting displacement, so crank stroke is pushed to the limit in terms of block height and rod length. For all practical purposes, there are no long-rod big-block Chevys other than 500-inch Pro Stock engines.
Wide LCA Syndrome
By this point, you should be convinced that you should be using cams with much tighter LCAs than found in a typical cam catalog. So let me now caution you about advice you may receive to the contrary.
If you order a cam with, for example, a 106-degree LCA by calling your favorite cam company, you may be told that having such a tight LCA cam for the street is not good for idle. Unfortunately, the cam tech guy has not thought things through and is just repeating what he was told. The idle quality is dependent on overlap, not on the LCA. If you choose a cam with too much overlap, the idle and, hence, street driving manners suffer.
For a longer cam, the only way to restore idle quality is to spread the LCA, which reduces the overlap. Thus it causes the output to drop everywhere in the RPM range. If maximizing power and torque are the goal, spreading the LCA to improve the idle and low-speed drivability is a very counterproductive move. The correct answer here is not to spread the LCA, but to go for less duration.
Fig. 9.9. A factory street 572 typically comes with a 112-degree LCA cam. Just to reinforce my point here, a cam for one of my street 525s, which normally has a 107-degree LCA cam, was installed. This 107-LCA cam was actually shorter than the 112 unit it replaced, and the overlap was less by 5 degrees. The 107-degree LCA cam closed the intake sooner and opened the exhaust later. Had this been on the 104- to 105-degree LCA cam needed for this engine, the differences would have been even more pronounced. This cam idled marginally smoother than the 112-degree LCA cam and the engine’s exhaust took on a much more authoritative tone.
Fig. 9.10. This graph shows distinct differences between the correct LCA for the displacement and valve size (in this case 107) versus a generic catalog cam with a 112 LCA. The difference in torque is almost the same as would be seen with the use of a stroker crank to make the engine into a 496.
To demonstrate this point, check out the dyno tests in Figures 9.10 and 9.11, where the effect of an LCA, as determined by Cam Master, and a typical catalog cam for a Chevy big-block at 112 degrees, are compared. The cams for the 572 are hydraulic rollers while those for the 468 are the more economically priced hydraulic flat-tappet style. Please take note of the fact that a properly spec’d and cheaper flat-tappet cam outperforms an incorrectly spec’d roller cam by a substantial margin.
Cam Timing Precision
The more accurately your cam is spec’d, the greater the potential for losing power if it is timed in wrong. Why is this so? Imagine an engine that has the exhaust events occurring close to where they should, but the intake events have been retarded. Advancing the cam makes the intake better but the exhaust worse. Some of the gains produced by the better intake timing are canceled by the worse exhaust. If the cam is optimally spec’d, the incorrect advance or retard makes both intake and exhaust worse as it moves off the optimal setting.
3 4 Racing Cam For A 454 Big Block Youtube Download
And that raises the question of what the optimal setting may be in terms of cam advance/retard. With a typical engine spec for heads and valvetrain, the 4-degree cam advance that the cam companies normally advise is nothing more than an educated guess for a typical engine. However, from the information in this book on what changes the optimal cam advance/retard settings, you will be in a far better position to make a judgment call if dyno testing to optimize is not possible.
First, make sure you understand what is meant by cam advance and retard (Figure 9.3). The easiest way to see the impact of various factors is to start with an optimal cam advance. As an example, let’s say you have a typical set of decent aftermarket heads, and the valvetrain employs 1.7:1 rockers.
With this setup, assume the optimal cam advance (found on the dyno) is 4 degrees. If you replace the intake rockers with ones that lift off the seat significantly faster, to the cylinder, the cam appears more advanced because the part of the overlap triangle delivered by the intake valve has increased. The cylinder also sees this as a slight closing of the LCA. With a previously optimal cam advance, going to faster off-the-seat rockers means retarding the cam. (The same situation occurs if you swap the heads for ones having much higher low-lift flow capability on the intake.)
The cam also needs to be retarded if you are testing rockers and go to a lower ratio on the exhaust. This sometimes pays off. The key issue with the exhaust is to reduce the cylinder pressure to about 35 psi or less at BDC on the exhaust stroke. With a big-block’s short rod/stroke ratio, the piston motion up the bore after BDC is very much slower than its motion away from TDC. In effect, the piston is hanging around the BDC position longer than would a long-rod/stroke ratio setup. This buys time for the cylinder to blow down even farther, and it allows the exhaust valve to get to a greater lift before the piston reaches any serious velocity up the bore.
Fig. 9.11. Figure 9.3 illustrates the effect of cam advance/retard. In these tests you see the effect of 0, 4, and 8 degrees of advance. The average torque with the cam set optimally at 4 degrees of advance was 645.4 ft-lbs. With the cam retarded 4 degrees, the average torque dropped to 640.2. With the cam advanced 4 degrees, the average torque was 636.6 ft-lbs. A point to note here is that this cam was on a 112-degree LCA, which was far removed from the 105-degree LCA needed for optimal valve events. If this cam had been correctly spec’d for this displacement, the consequences of incorrect cam timing would be two to three times greater than is seen here.
Another example here serves to demonstrate why the usual 4-degree advance recommendation is just that, a recommendation. If the intake valveseat has been cut so that it produces a significantly better low-lift flow then, from the cylinder’s point of reference, it appears that the cam has been advanced.
To further reinforce this point, let’s use an example with the trick valveseats I cut on my heads. With a 4-degree advance, the cylinder would think that the cam was 3 to 5 degrees more advanced than it actually is. Also, the LCA angle could appear to be up to 2 degrees tighter than they actually are. (Therefore, flow figures are better than valve sizes for selecting an LCA.) To compensate, the cam must be retarded.
Here is an example. The cam card called for the typical 4 degrees of advance and that is how the cam was installed. However, the heads had one of my trick valveseat jobs and, in the low-lift range, flowed 12 percent better than average. With the high-flow seats, the optimum, as was quickly found with the Jesel belt drive, was established by the dyno results to be 1-degree retarded. The difference in output was 25 hp!
3 4 Racing Cam For A 454 Big Block Youtube Channel
There are two facts to take in here. First, low-lift flow positively contributes to a higher output. Second, higher output isn’t realized unless the cam advance/retard situation is fully explored, and that means having an adjustable cam drive.
Valve Lift Considerations
Because of the Chevy big-block’s architecture, the valve lift needed for anything other than a valvetrain with a long-duration big-journal race cam plus big-diameter lifters cannot be achieved. For a 496-ci engine with 2.3 intake valves and a 270-degree cam, lift needs to be in the 0.900-inch range. Such an amount of lift would be almost impossible with typically available parts. These engines require very high lift for any given duration, but at shorter durations there is almost no chance of achieving any-thing near what the engine wants for optimal results. So you must strike compromises.
Determining the lift needed for a target output and getting it are very much tied to parts selection. Chapter 10, Valvetrain Optimization, discusses both topics so that the end result is the best bang for your buck.
Written by David Vizard and Posted with Permission of CarTechBooks
GET A DEAL ON THIS BOOK!
If you liked this article you will LOVE the full book. Click the button below and we will send you an exclusive deal on this book.